
The Placula hypothesis Resurected
If you've never heard of placozoans, you're not alone.2 These small animals have been known for more than a century, but for a long time were dismissed as aberrant forms of cnidarian larvae.4
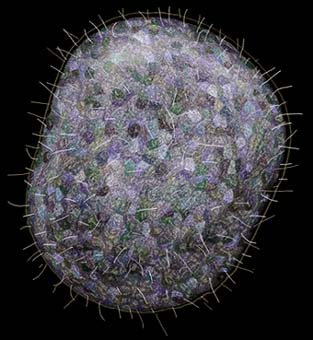
Figure 1: Artist's rendering of a placozoan. (by Wim van Egmond using Adobe Photoshop.)
However, these animals are at the center of a tangled set of mysteries regarding the beginnings of the animals. There are two recent papers,2, 6 describing a new, or rather newly reconstituted, theory of how the animals evolved and differentiated.
Blackstone, N. (2009). A New Look at Some Old Animals PLoS Biology, 7 (1) DOI: 10.1371/journal.pbio.1000007
Schierwater, B., Eitel, M., Jakob, W., Osigus, H., Hadrys, H., Dellaporta, S., Kolokotronis, S., & DeSalle, R. (2009). Concatenated Analysis Sheds Light on Early Metazoan Evolution and Fuels a Modern “Urmetazoon” Hypothesis PLoS Biology, 7 (1) DOI: 10.1371/journal.pbio.1000020
I recently discussed the Urbilaterians, the original ancestor of all bilateria. But there are other "Ur's" to be considered, specifically the Ureumetazoans (the ancestor of all eumetazoans), and the Urmetazoans (the original ancestor of all animals). The precise description of these three ancestors is a well-debated subject.
The thrust of these two papers is to resurect a long-defunct hypothesis regarding what the original animal, the Urmetazoan, was like: the Placula hypothesis. The basic idea is that the Urmetazoan, the common ancestor of all animals, looked very much like a placozoan. Adaptive radiation would then have created the various major lineages from there.
Figure 2: The Placula Hyphothesis. Click to see full picture and original caption. (From reference 6.)
The primary justification for this resurrection is the publication of several phylogenetic trees that place the bilaterians at a position basal to the entire metazoans: as a sister group to the combined clade of placozoans, sponges, and cnidarians.
Figure 3: Phylogenetic tree showing the bilaterians as basal to the metazoans. Click to see full picture and original caption. (From reference 6.)
Problems With the Placula Hyphothesis
There are, however, some problems with this. For instance, three previous references28, 29, 30 found this relationship but dismissed as due to long branch attraction, one of the problems that plague this sort of analysis.
Another issue is the number of shared genetic features among the bilaterians, the cnidarians, and the sponges that would have to all have been lost by the placozoans. Just as one example, many developmental genes show a much closer relationship between the bilaterians and the cnidarians.23

Figure 4: Phylogeny of animals and their protistan allies based on the combined SEAß nt sequences. Click to see original caption. (From Reference 23.)
Another problem is the shared use of "collared flagella" by the choanoflagellates and the sponges. If even the placozoans had branched off in-between these two groups, an explanation is needed for why they lack this feature. Having the bilaterians also branch in-between makes the problem much worse.
What makes the placula hypothesis particularly difficult is that it doesn't just require these branchings, but assumes that the urmetazoan, the original animal, has lost the collared flagella it presumably could have inherited from the common ancestor of animals and choanoflagellates, then the sponges regained it.
A Better Hypothesis (IMO)
A better hypothesis is that the urmetazoan used collared flagella, just like the choanoflagellates, but that the placozoans have since lost this feature. Here's how I see it:
The original animal was somewhat like a larval sponge: a small ball of cells, but with differentiation and differential expression of a bunch of genes associated with developmental control in almost all animals.23, 24, 25, 26
Figure 5: Expression of Wnt, a developmental gene, in Amphimedon (a sponge) embryos. Click on picture to see original caption. (From reference 24.)
Like sponges and choanoflagellates, it used a collared flagellum for its food, and like sponge larvae it could swim and possessed phototaxis and chemosensing abilities, able to guide its swimming based on external sensory information.25
I made the argument in Searching for the Urbilaterian that no matter what symmetry an animal has, or not, it has to know its left from its right, and its up from its down, in order to guide its activity. The same is true for phototaxis. Thus, even the larvae of sponges must have some genes differentially expressed to let cells know where they are in the body. The same almost certainly holds true for placozoans, which also have differentially expressed genes, although none have yet been proven to provide lateral directionality.15, 16, 17
This has important implications for the urmetazoan. If my theory is correct, this little animal fed like a sponge, but with only a small number of collared flagella on the front of its ball-like shape. It would have had extra cilia for swimming and steering, at least as much sensory ability as placozoans and larval sponges, and a basic suite of developmental genes and signaling molecules. It would have had radial symmetry (like a sponge larva), but this would have been superficial, overlaid on a gene expression pattern that resolved all the ambiguities of location for each cell, allowing complete coordination between senses and swimming.
This animal would not have had nerves, however it may well have had a syncytial network (an extensive region of multinucleate cytoplasm) serving the functions of both nerves and muscles, something possessed by the extremely simple placozoans, as well as by one group of sponges: the Hexactinellids. Communication would have been by chemical signals, something seen in sponges.27
Such an animal would be perfectly positioned to evolve into sponges, placozoa, and eumetazoans. It would have the feeding mechanisms, inherited from the common ancestor of animals and choanoflagellates, that it would pass on to the sponges. It would have a simple body plan that, by flattening and specializing, could create the placozoans. That same body plan, by inverting one half of its skin into a hollow hemisphere, would create a gastrula-like creature not too much different from a simple cnidarian or ctenophore.

Figure 6: development of a gastrula from a blastula. (From Wiki.)
Most likely this creature had some ability to modify its body shape, as is seen in the placozoans. The syncytial network in these creatures can make extreme modifications to its shape,1 and there's no reason to suppose the urmetazoan couldn't do likewise.
Multicellularity
This animal could have easily evolved from a single-celled ancestor, however I have another idea. Could it be that the common ancestor of fungi and animals was actually multinucleate, an amoeba-like creature with lots of nuclei, a flexible shape, and a feeding pattern based on engulfing its food?
Such a creature would be well positioned to evolve into both fungi and metazoans, with the latter branch having lots of collared flagelli. The question is, why evolve multiple cells? The answer could well be an explosive adaptive radiation of invasive, intracellular predators. Such an explosion would explain the sudden acquisition of multicellularity by many lineages.
The most important thing would have been to develop a layer of mononucleate cells on the surface, capable of rapid self-destruction if successfully invaded. The internal parts could remain multinucleate, something that is at least somewhat true for a large variety of animal lineages today. Even humans have multinucleate cells, specifically our muscles.
But for many lineages, the answer would have been to go to independent singly nucleated cells. Thus, it's possible that many lineages that, today, are split into multicelled and single-celled groups are descended from earlier lineages that were technically single cells, but with many nuclei.
Links: (These aren't in any particular order, and all of them may not be called out in the text. Use the back key if you got to one by clicking a footnote.)
1. My favorite animal, Trichoplax adhaerens
2. A New Look at Some Old Animals
3. Field biology of placozoans (Trichoplax): distribution, diversity, biotic interactions
4. Trichoplax adhaerens: Discovered as a missing link, forgotten as a hydrozoan, re-discovered as a key to metazoan evolution
5. Phylogenetic Context and Basal Metazoan Model Systems
6. Concatenated Analysis Sheds Light on Early Metazoan Evolution and Fuels a Modern “Urmetazoon” Hypothesis
7. Key transitions in animal evolution
8. A food's-eye view of the transition from basal metazoans to bilaterians
9. Implications of cnidarian gene expression patterns for the origins of bilaterality—is the glass half full or half empty?
10. Key transitions in animal evolution: a mitochondrial DNA perspective
11. Key transitions during the evolution of animal phototransduction: novelty, "tree-thinking," co-option, and co-duplication
12. Evolution of the bilaterian germ line: lineage origin and modulation of specification mechanisms
13. The Trichoplax genome and the nature of placozoans
14.Non-coding RNA annotation of the genome of Trichoplax adhaerens
15.The Trox-2 Hox/ParaHox gene of Trichoplax (Placozoa) marks an epithelial boundary
16.The Early ANTP Gene Repertoire: Insights from the Placozoan Genome
17.The Trichoplax PaxB Gene: A Putative Proto-PaxA/B/C Gene Predating the Origin of Nerve and Sensory Cells
18.Lower Cambrian Vendobionts from China and Early Diploblast Evolution (Requires free registration)
19.Are Hox Genes Ancestrally Involved in Axial Patterning? Evidence from the Hydrozoan Clytia hemisphaerica (Cnidaria)
20.The Protistan Origins of Animals and Fungi
21.Molecular phylogeny of choanoflagellates, the sister group to Metazoa
22.Multigene Phylogeny of Choanozoa and the Origin of Animals
23.Hox, Wnt, and the evolution of the primary body axis: insights from the early-divergent phyla
24.Wnt and TGF-β Expression in the Sponge Amphimedon queenslandica and the Origin of Metazoan Embryonic Patterning
25.Developmental expression of transcription factor genes in a demosponge: insights into the origin of metazoan multicellularity
26.Emergence, development and diversification of the TGF-β signalling pathway within the animal kingdom
27.Neuroactive substances specifically modulate rhythmic body contractions in the nerveless metazoon Tethya wilhelma (Demospongiae, Porifera)
28.Mitochondrial genome of Trichoplax adhaerens supports Placozoa as the basal lower metazoan phylum
29.Mitochondrial Genomes of Two Demosponges Provide Insights into An Early Stage of Animal Evolution
30.Comparative Genomics of Large Mitochondria in Placozoans
What an interesting post!
ReplyDeleteFor some time, I have been ruminating over what sort of evolutionary pressure would lead cells to become multinucleated, when in fact, as I now realise, the converse question might be better motivated. (It's not easy to snap out of the mononuclear norm when you're a human biologist!)
There is a somewhat obscure literature suggesting that the "smallest unit of life" is not the cell, in eukaryotic organisms, but rather the cell nucleus with its associated organelles. In this view, the cell membrane and cytoplasm are in a way a constructed habitat rather than integral parts of the organism. See for example Baluska et al, Annals of Botany 94: 9-32, 2004.
In fact, I have gathered some data on the regulation of gene expression in multinucleated human cells suggesting a high degree of functional independence between the nuclei. We'll see where that goes, it's too early to draw any conclusions yet, but it's possible that we have to rethink our concepts and models a bit.
Glad you liked it.
ReplyDeleteThanks for the reference. The link, for anyone interested, is Eukaryotic Cells and their Cell Bodies: Cell Theory Revised.
What I'd like to do is question the whole idea of the "smallest unit of life". Life is based on evolution, that is natural selection. Even the multicelled individual is little more than a robot expressing its genes for natural selection to act on. Given this, why does there need to be a unit of life in a fundamental sense?
Good point.
ReplyDeleteI too subscribe to the "selfish gene" idea.
Nevertheless, a replicator on its own - a single RNA molecule, for example - is capable of reproduction but not of any of the other features we associate with life, such as metabolism or organisation. The question where the system has such dignity as to deserve to be called "alive" is semantic and the answer is arbitrary.
If we want to give the concept of "life" a meaning, then there must be a limit somewhere between that which lives and that which doesn't. I think these notions influence the mental models we have of biological systems, and frankly it gets me excited even though I'm aware that it's just an arbitrary taxonomy we are sticking on to the biology.
I agree, but I think we have to be careful about being too reductionist with concepts that are somewhat holistic in nature. I'd group "life" in that class.
ReplyDeleteConsider the mitochondria, for instance: The "single RNA molecule" that is a mitochondrial genome is capable of replication, but (in most types of cell) the mitochondrion is not.
Similarly, nuclear DNA replication can be decoupled from cellular replication in a variety of ways (other than simple multi-nucleated cells): consider the micro- and macro-nuclei of ciliates, for instance. And the duplication of parts of the genome in specific somatic cells in growing animals (e.g. Drosophila).
Having read the paper you mentioned, I'm going to have to think about it a while, and read some of the references, but my immediate reaction is that it's too DNA-centric: it's the total organization of components that performs the replication, the "DNA=master, rest=slave" notion seems simplistic to me. That's a bit like saying the CDs in my shelf are the masters and I'm the slave when I get them out and put them in the player.